🧫 Chemical Truth from the Field of Master Mind Chemistry (MMCHEM) 🔬
Shared from the research of: Joseph Mercado 👨🔬
Article Authors: Natalie Wiedemar, Dennis A. Hauser, and Pascal Mäser 👩⚕️🧑⚕️👨⚕️
Content Contributor: National Library of Medicine 🌏
To: Health Analyst 🌿
Blog Post #1217 📌
Re: 100 Years of Suramin 🌲
Date and Time: Thursday, April 21, 2022 at 3:24 p.m. ⏰
Dear Health Analyst,
Are you aware of the powerful effects of Suramin?
Let’s dissect it under a microscope for just moment…
ABSTRACT:
Suramin is 100 years old and is still being used to treat the first stage of acute human sleeping sickness, caused by Trypanosoma brucei rhodesiense.
Suramin is a multifunctional molecule with a wide array of potential applications, from parasitic and viral diseases to cancer, snakebite, and autism.
Suramin is also an enigmatic molecule: What are its targets? How does it get into cells in the first place?
Here, we provide an overview of the many different candidate targets of suramin and discuss its modes of action and routes of cellular uptake.
We reason that, once the polypharmacology of suramin is understood at the molecular level, new, more specific, and less toxic molecules can be identified for the numerous potential applications of suramin.
SURAMIN, THE FRUIT OF EARLY MEDICINAL CHEMISTRY:
When suramin was introduced for the treatment of African sleeping sickness in 1922, it was one of the first anti-infective agents that had been developed in a medicinal chemistry program.
Starting from the antitrypanosomal activity of the dye trypan blue, synthesized in 1904 by Paul Ehrlich, Bayer made a series of colorless and more potent derivatives.
Molecule 205 was suramin (Fig. 1), synthesized by Oskar Dressel, Richard Kothe, and Bernhard Heymann in 1916.
Sleeping sickness (also known as human African trypanosomiasis [HAT]) was at the forefront of research at that time, not a neglected disease as it is today, and the development of suramin was a breakthrough for the emerging field of chemotherapy.
While the history of suramin has been reviewed elsewhere, we focus here on the many potential applications of suramin and its enigmatic mode of action.
SURAMIN AS AN ANTIPARASITIC DRUG:
Suramin is still being used for the treatment of Trypanosoma brucei rhodesiense infections.
However, it does not cross the blood-brain barrier and therefore is administered only for the first (hemolymphatic) stage of sleeping sickness, when the trypanosomes have not yet invaded the patient’s central nervous system (CNS).
The standard treatment regimen for suramin is an initial test dose of 4 to 5 mg/kg of body weight followed by five weekly doses of 20 mg/kg (but not more than 1 g) injected intravenously (i.v.)
Suramin is also used for surra (mal de caderas), caused by Trypanosoma evansi, in particular for the treatment of camels.
The treatment regimen is a single i.v. injection of 10 mg/kg suramin, i.e., about 6 to 10. In vitro, suramin also has some activity against Trypanosoma cruzi.
However, it is not used for Chagas’ disease, and studies in mice have even suggested that suramin would exacerbate the disease.
In vitro activity of suramin against Leishmania major and Leishmania donovani has recently been described.
Furthermore, suramin blocks host cell invasion by the malaria parasite Plasmodium falciparum.
This was observed for both the invasion of erythrocytes by P. falciparum merozoites and the invasion of HepG2 hepatoma cells by P. falciparum sporozoites.
Suramin had been in use for river blindness, caused by the filarial parasite Onchocerca volvulus.
It acts both on microfilariae and, to a greater extent, on adult worms.
However, suramin was subsequently replaced by the less toxic, and orally bioavailable, ivermectin.
The adverse effects of suramin are indeed manifold, including nephrotoxicity, hypersensitivity reactions, dermatitis, anemia, peripheral neuropathy, and bone marrow toxicity.
However, despite its potential toxicity, the lack of bioavailability, and the absence of lead-like properties (Fig. 1), suramin has found a surprising variety of repurposing applications.
Table 1 provides an overview of the biological activities of suramin, and Table 2 lists clinical trials performed with suramin.
SURAMIN AS AN ANTIVIRAL AGENT:
The antiviral and antibacteriophage activities of suramin have been known since the mid-20th century.
Soon after the discovery of retroviruses, suramin was found to inhibit retroviral reverse transcriptase, which served as a rationale to test suramin against human immunodeficiency virus (HIV).
Suramin protected T cells from HIV infection in vitro (19), and in AIDS patients, it reduced the viral burden in some of the study subjects; however, no improvement of the immunological features and clinical symptoms was achieved.
Later, suramin was found to inhibit host cell attachment through binding to the HIV-1 envelope glycoprotein gp120, indicating that the in vitro protection against HIV infection is mediated through inhibition of viral entry.
Suramin also inhibits the binding of dengue virus to host cells through a direct effect on the viral envelope protein.
Inhibition of host cell attachment was also found for herpes simplex and hepatitis C viruses, which explained the previously reported protective effects of suramin against in vitro herpes simplex virus infections and in vivo infections of ducks with duck hepatitis B virus.
Similar to the experience with HIV, suramin had been initially tested against hepatitis viruses due to its inhibitory effect on the viral DNA polymerase.
However, in a small clinical trial, suramin was found to be ineffective and toxic in chronic active hepatitis B patients.
Suramin neutralized enterovirus 71 (EV71) in cell culture and in a mouse model by binding to capsid proteins.
Suramin also has potential against emerging viruses.
It was shown to inhibit both RNA synthesis and replication in chikungunya virus.
In vitro, suramin conferred protection if present at the time of infection, and this was attributed to a reduction of viral host cell binding and uptake.
In the murine model, suramin led to a reduction of pathognomonic lesions if injected prior to chikungunya virus infection.
Suramin also inhibited host cell invasion by Ebola virus and Zika virus, even when added after viral exposure of the cell cultures.
SURAMIN AGAINST CANCER:
The first studies on the effects of suramin on neoplasms in animals were carried out in the 1940s; mice engrafted with lymphosarcoma developed significantly smaller tumors when simultaneously treated with suramin.
In the 1970s, it was shown that suramin could enhance the actions of cyclophosphamide and adriamycin in mice engrafted with Ehrlich carcinoma.
The first clinical trial with suramin was carried out in the 1980s in advanced-stage adrenal and renal cancer patients. Around half of the patients showed either partial or minimal responses, and none showed complete remission.
Nevertheless, a number of subsequent clinical trials with suramin were carried out (Table 2). In particular, suramin was tested against prostate cancer, non-small cell lung cancer, breast cancer, bladder cancer, and brain tumors.
Most of the studies were based on the potential of suramin to act as an antagonist of growth factors, which are often overexpressed by tumors. In addition, suramin directly exhibits cytostatic activity on cultured tumor cells.
However, the initial clinical tests did not warrant the further development of suramin as an anticancer monotherapy.
Subsequent tests focused on suramin as a chemosensitizer, based on the findings that, at subcytotoxic levels (<50 μM), it enhanced the efficacy of anticancer drugs, such as mitomycin C, taxol, or doxorubicin, in ex vivo cultures and in animal models (63,–65). Suramin combined with taxol inhibited invasiveness and prevented metastasis in a xenograft mouse model.
Different explanations are conceivable for the chemosensitizing effects of suramin on tumor cells, including inhibition of telomerase (67) or inhibition of fibroblast growth factors and angiogenesis.
A phase II clinical study was performed in patients with advanced, drug-resistant non-small cell lung cancer treated with taxol or carboplatin; supplementation with nontoxic doses of suramin did not overcome drug resistance.
Randomized controlled studies to validate the use of suramin as a chemosensitizer in chemotherapy-naive lung cancer patients remain to be performed. A combination of estramustine, docetaxel, and suramin gave promising results in hormone-refractory prostate cancer patients.
SURAMIN AS AN ANTIDOTE:
Three of the many biological activities of suramin support its potential use as a protective agent: the inhibition of thrombin, the inhibition of phospholipase A2, and the inhibition of purinergic signaling. Several vipers possess toxins that mimic thrombin, perfidiously triggering the coagulation cascade in mammalian blood.
Suramin not only inhibits thrombin itself, but also the thrombin-like proteases of snake venom, and was therefore proposed as an antidote for snakebite.
Other common constituents of metazoan venoms are phospholipases A2, which convert phospholipids into lysophospholipids.
Again, suramin inhibits mammalian phospholipase A2, as well as the orthologues from snake venom and bee venom, suggesting that it can act as an antidote.
A certain degree of protection from venoms by suramin was confirmed in mouse models.
The potential use of suramin as an antidote is attractive, given the high global burden of snakebites and the current shortage of antivenom.
Suramin’s ability to block P2 purinergic, G protein-coupled receptors may counteract the action of neurotoxins that trigger arachidonic acid signaling, e.g., via phospholipase A2 activity.
A possible explanation is that suramin prevents the activation of ATP receptors at the motor nerve ending, which otherwise would depress Ca2+ currents and reduce acetylcholine release at the presynaptic membrane.
Suramin was also proposed to serve as a neuroprotective agent and as an antidote for kidney toxicity during cancer chemotherapy and, based on its antiapoptotic effect, to protect against liver failure.
Suramin also inhibits connexin channels of the tight junction, thereby suppressing ATP release and protecting cells from pore-forming bacterial toxins, such as hemolysin.
The suramin analogs NF340 and NF546 were cardioprotective in a mouse model for heart graft rejection, presumably via inhibition of the purinergic G protein-coupled receptor P2Y11.
FURTHER POTENTIAL USES OF SURAMIN:
Suramin was found to have beneficial effects in a rat arthritis model and to suppress fear responses in the rat. It also promoted the expansion of T cells during immunization of mice and was therefore considered as a small-molecule adjuvant for vaccination.
Based on the cell danger hypothesis, suramin has recently been tested for the treatment of autism spectrum disorders (ASD).
The cell danger hypothesis suggests that a systemic stress response that involves mitochondria and purinergic signaling contributes to the development of psychopathologies like autism. Suramin had been shown to act as an inhibitor of purinergic signaling and mitochondrial function and was therefore proposed as a potential therapy for ASD.
First tests in mouse models showed correction of symptoms in juveniles, as well as in adults.
A first small human trial was carried out and, even though difficult to quantify, showed improvement of ASD symptoms.
TOO MANY TARGETS:
Suramin is a large molecule that carries six negative charges at physiological pH (Fig. 1).
It is likely to bind to, and thereby inhibit, various proteins.
Thus, the many and diverse potential applications of suramin reflect its polypharmacology.
Indeed, a large number of enzymes have been shown to be inhibited by suramin (Table 3).
Suramin inhibits many glycolytic enzymes, enzymes involved in galactose catabolism (PubChem BioAssay no. 493189), and enzymes of the Krebs cycle.
Suramin further decreases the activities of a large number of enzymes involved in DNA and RNA synthesis and modification: DNA polymerases, RNA polymerases, reverse transcriptase, telomerase, and enzymes involved in winding/unwinding of DNA are inhibited by suramin, as well as histone- and chromatin-modifying enzymes like chromobox proteins, methyltransferases, and sirtuin histone deacetylases.
Suramin is also an inhibitor of other sirtuins and protein kinases, glutaminase (PubChem BioAssay no. 624170), phospholipase A2, protein tyrosine phosphatases, lysozyme, and different serine and cysteine proteases.
For caspases, cysteine proteases involved in apoptosis, suramin was described as acting as either inhibitor or activator.
Suramin further inhibits the Na+,K+-ATPase and other ATPases, certain classes of GABA receptors, and several G protein-coupled receptors, including P2 purinoceptors and follicle-stimulating hormone receptor.
Suramin also showed inhibitory effects against components of the coagulation cascade and the complement system and against deubiquitinating enzymes (PubChem BioAssay no. 504865 and 463106).
It also interacts with prion protein, inhibiting conversion into the pathogenic form PrPSc.
Besides its many inhibitory activities, suramin also activates certain nuclear receptors that act as transcription factors and intracellular calcium channels.
ENIGMATIC MECHANISMS OF ACTION AGAINST AFRICAN TRYPANOSOMES:
Somewhat ironically, much less appears to be known about the targets of suramin in African trypanosomes, where it has been in use for a century, than those in tumor cells or viruses.
Suramin was shown to inhibit glycolytic enzymes of T. brucei, with selectivity over their mammalian orthologues, in particular, hexokinase, aldolase, phosphoglycerate kinase, and glycerol-3-phosphate dehydrogenase. Intriguingly, the trypanosomal enzymes have higher isoelectric points (>9), which is due to extra arginines and lysines that are absent in the mammalian orthologues.
These residues form positively charged surface-exposed “hot spots” that were proposed to be bound by the negatively charged suramin.
Inhibition of trypanosomal glycolysis by suramin is in agreement with the dose-dependent inhibition of oxygen consumption and ATP production observed in trypanosomes isolated from suramin-treated rats.
However, the glycolytic enzymes of T. brucei are localized inside glycosomes, and it is unclear how suramin could penetrate the glycosomal membrane or if suramin could bind to glycolytic enzymes in the cytosol before they were imported into glycosomes.
Alternative targets proposed for the trypanocidal effect of suramin are glycerophosphate oxidase (141, 142); a serine oligopeptidase termed OP-Tb; and REL1, the RNA-editing ligase of the trypanosome’s kinetoplast.
It is unclear how suramin would pass the inner mitochondrial membrane, but suramin inhibited oxidative phosphorylation in mitochondrial preparations of the trypanosomatid Crithidia fasciculata.
Suramin also appeared to inhibit cytokinesis in T. brucei, as indicated by the finding that suramin treatment resulted in an increased number of trypanosomes with two nuclei (146).
UPTAKE ROUTES OF SURAMIN INTO CELLS:
The negative charges of suramin (Fig. 1) not only promote binding to various proteins, they also prevent diffusion across biological membranes. However, the majority of targets (Table 3) are intracellular, and radiolabeled suramin was shown to be taken up by human endothelial and carcinoma cells and by T. brucei bloodstream forms.
Suramin is not a substrate of P-glycoprotein or of any other known transporter.
Thus, suramin must be imported by endocytosis. Mammalian cells can take up suramin in complex with serum albumin by receptor-mediated endocytosis.
This had originally also been thought to happen in T. brucei.
However, the trypanosomes do not take up albumin by receptor-mediated endocytosis (151), and LDL (low-density lipoprotein) was proposed to act as the vehicle instead. Suramin bound to LDL and inhibited the binding and uptake of LDL, while LDL enhanced the uptake of suramin in bloodstream form T. brucei.
In contrast, overexpression in procyclic T. brucei of Rab4, a small GTPase involved in the recycling of endosomes, decreased suramin binding and uptake without affecting LDL binding or uptake. In the same study, overexpression of a mutant Rab5, which was locked in the active GTP-bound form, increased LDL uptake without affecting suramin uptake.
These findings indicated that, at least in the procyclic trypanosomes of the tsetse fly midgut, LDL and suramin are imported independently of each other.
The development of genome-wide RNA interference (RNAi) screens in bloodstream form T. brucei combined with next-generation sequencing offered new opportunities to address the genetics of drug resistance.
This approach identified genes whose silencing reduced sensitivity to suramin (153). They included a number of genes encoding endosomal and lysosomal proteins, in agreement with uptake of suramin through endocytosis.
The invariant surface glycoprotein ISG75 was identified as a likely receptor of suramin, since knockdown of ISG75 in bloodstream form T. brucei decreased suramin binding and suramin susceptibility. ISG75 is a surface protein of unknown function whose abundance is controlled by ubiquitination.
Thus, there appear to be (at least) two pathways for receptor-mediated endocytosis of suramin in T. brucei bloodstream forms: either directly, with ISG75 as the receptor, or after binding of suramin to LDL, together with the LDL receptor.
CONCLUSIONS:
Suramin remains controversial.
Is its polypharmacology a liability or an asset?
Is it toxic or protective? Dated or timeless?
Whatever the verdict on suramin, there is hardly another molecule with as many biological activities.
The list of potential targets is indeed impressive, and the publication stream on suramin is not stagnating.
The large majority of papers are not about trypanosomes or trypanosomiasis (Fig. 2).
The list of potential targets has to be taken with a grain of salt, though, since the negative charges of suramin, and its promiscuity in protein binding, can cause all kinds of artifacts.
Suramin can dissolve Matrigel, resulting in a false-positive signal in cell-based screening campaigns that use Matrigel for support, e.g., for inhibitors of angiogenesis.
On the other hand, suramin’s high affinity for albumin may give false-negative results in cell-based tests that contain mammalian serum.
However, in spite of the various confounders, a number of different drug-target interactions for suramin have been experimentally validated and are directly supported by crystal structures (Table 4).
Several routes of investigation of the bioactivities of suramin have culminated in clinical trials with healthy volunteers (i.e., phase I) or patients (i.e., phases II and III) (Table 2).
However, to our knowledge, none of these trials was a striking success, and it is unclear whether suramin will ever find medical applications outside the field of parasitology.
However, molecules that act similarly to suramin may be identified via target-based screening once the mode of action is understood—new molecules that are more specific and less toxic and possess better pharmacological properties than suramin.
Thus, it will be important to dissect the polypharmacology of suramin at the molecular level. We hope that the compiled list of targets (Table 3) will serve this purpose.
Content Source: NCBI 🌍
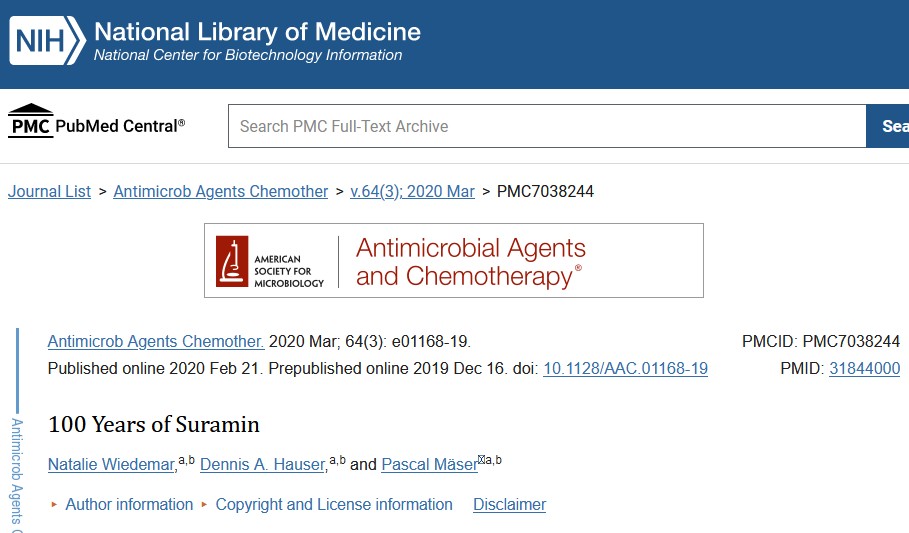
Email Us a Message 
Have a question about this article
Please send us a personal message below and we will serve you momentarily.